“This research sheds new light on the nature of dark matter and its distribution in the Milky Way.”
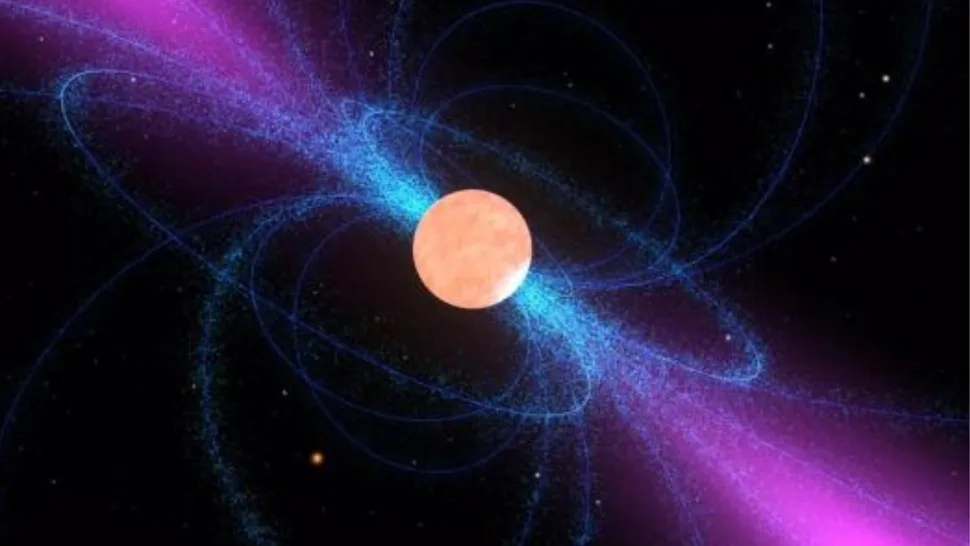
Scientists are set to use dead star “clocks” to illuminate one of the universe’s most enigmatic phenomena: dark energy.
These cosmic timekeepers, known as pulsars, are rapidly spinning neutron stars formed when stars at least eight times as massive as the sun die. The extreme conditions of neutron stars make them ideal laboratories for studying physics in environments found nowhere else in the universe.
Millisecond pulsars, a type of pulsar, can spin hundreds of times per second, emitting beams of electromagnetic radiation from their poles like cosmic lighthouses. Initially, these neutron stars appeared to be pulsing, increasing in brightness when their beams were directed at Earth.
The ultraprecise timing of millisecond pulsars’ brightness variation means they can be used collectively as cosmic timepieces in “pulsar-timing arrays.” These arrays are so precise they can measure gravitational disturbances in the fabric of space and time, united as a four-dimensional entity called “spacetime,” which could be the ideal way of hunting dark matter.
“Science has developed very precise methods to measure time,” said pulsar timing array researcher John LoSecco from the University of Notre Dame. “On Earth, we have atomic clocks, and in space, we have pulsars.”
Unraveling the Dark Matter Mystery
Dark matter remains one of the most mysterious substances in the universe because it doesn’t interact with light or ordinary matter — or if it does, it does so very weakly, making it undetectable. Ordinary matter, made up of atoms with electrons, protons, and neutrons, interacts with light and matter, so scientists know dark matter must be composed of different particles.
Despite not interacting with light, dark matter does have a gravitational influence. This presence can be inferred when its gravitational effects alter the behavior of light and ordinary matter. LoSecco and colleagues aimed to exploit this gravitational influence using pulsars.

According to Albert Einstein’s theory of general relativity, objects with mass curve the very fabric of spacetime, and gravity arises from this curvature. When light passes through this curvature, its path is diverted, changing the travel time of light. This means light from the same distant body can arrive at Earth at different times, theoretically “slowing it down” (the speed of light remains constant, but the distance it travels changes).

Dark matter has mass and can warp spacetime, too. Thus, light from distant objects is curved, and its arrival time is delayed when it passes through concentrations of dark matter. This effect, known as “gravitational lensing,” involves intervening bodies acting as “gravitational lenses.”
LoSecco and his team examined data collected from 65 pulsars in the Parkes Pulsar Timing Array. They observed around 12 incidents indicating variations and delays in the pulsars’ timings, which usually have nanosecond accuracy.
This suggests that the radiowave beams from these cosmic lighthouses are traveling around a warp in space caused by an unseen concentration of mass between the pulsar and the telescope. The team theorizes these invisible masses could be dark matter “clumps.”
“We take advantage of the fact that the Earth, the sun, the pulsar, and even the dark matter are all moving,” LoSecco explained. “We observe deviations in arrival times caused by changes in distance between the mass we are observing and the line of sight to our ‘clock’ pulsar.”
The deviations observed are minuscule. For example, a body with the mass of the sun would cause a delay in pulsar radiowaves of about 10 microseconds. The proposed dark matter delay deviations seen by the team are 10,000 times smaller than that.
“One of the findings suggests a distortion of about 20% of the mass of the sun,” LoSecco said. “This object could be a candidate for dark matter.”
Improving Data Precision
A side effect of the team’s research is the improved precision of the Parkes Pulsar Timing Array data, collected to search for evidence of low-frequency gravitational radiation. Dark matter conglomerations can add interference or “noise” to this data. Identifying and removing that noise will help scientists better utilize this sample set in searches for low-frequency gravitational waves. This could enable the detection of gravitational radiation from more distant and earlier black hole mergers — and perhaps even background primordial gravitational waves left over from the Big Bang.
“The true nature of dark matter is a mystery,” LoSecco said. “This research sheds new light on the nature of dark matter and its distribution in the Milky Way and may also improve the accuracy of precision pulsar data.”
The team’s results were presented at the National Astronomy Meeting (NAM) 2024 at the University of Hull on Monday, July 15.